Current Student Research Projects
Investigator: Autumn Rasmussen
Email: arasmussen8@wisc.edu
Member of UWMRRC since 2021
Historically, broad beam gamma-ray sources such as 60Co and 137Cs were used in radiobiology. This was beneficial as the known photon energies from the sources in the Compton scattering energy range when used for whole-body irradiation yielded consistent results. Some divergence from this method can be attributed to policies implemented by the United States government in the early 2000s which were designed to prevent the misuse of radioactive isotopes. Furthermore, deviation from broad beam techniques to smaller fields is more applicable to a clinical setting. For these reasons, there has been a widespread replacement of gamma-ray irradiators with orthovoltage x-ray machines which have the benefit of being safer, cost-effective, and self-shielding.
This project aims to enhance traceability and set new standards for dosimetry in radiobiology by focusing on two main objectives. First, it will characterize a low dose rate 137Cs irradiator and validate in vivo dosimetry using TLD microcubes implanted in mice. Second, the project will characterize x-ray beams in radiobiological cabinet and conformal irradiators, leading to the establishment of UW Radiobiology-beams for calibration and uncertainty budgets with traceable comparisons to primary standards.
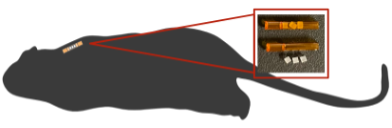
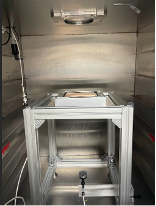
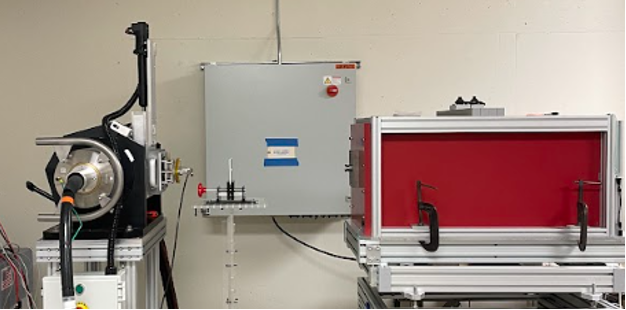
Investigator: John Stasko, M.S.
Email: jstasko@wisc.edu
Member of the calibration lab since 2019
Historically, mammography units have used molybdenum-anode x-ray tubes. However, since the late 1990s, tungsten-anode x-ray tubes have become more popular. Now, the majority of modern mammography units use tungsten-anode x-ray tubes. In Europe and other parts of the world, calibration laboratories have implemented tungsten-anode calibration beams for mammographic energies. Calibration laboratories in the US have not caught up with this trend, as no tungsten-anode mammography calibration beams exist in this country.
My project involves developing tungsten-anode mammography calibration beams at UW, matched in terms of half-value layer with beams in development at NIST. To do this, I have been running simulations and taking measurements using various devices such as a free-air chamber, ionization chambers, and a CdTe spectrometer (shown in the picture below). Once the beams are fully-characterized, I can use them to evaluate the performance of x-ray multimeters, solid-state dosimeters that claim to make diagnostic imaging dosimetry simple. The end goal of my project is to improve the state of mammographic dosimetry in the US, in order to better understand the potential risks that these procedures have on the patient.
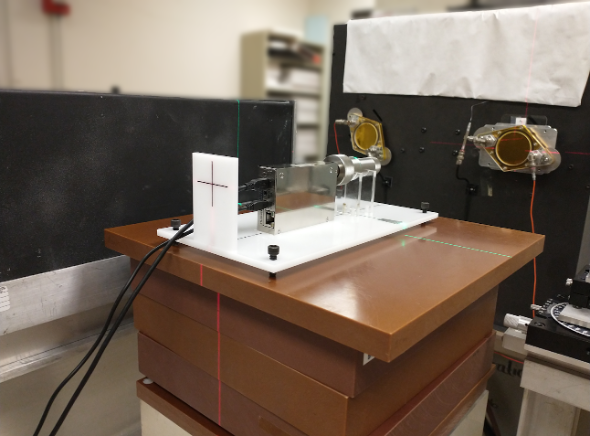
Investigator: Miguel Angel Flores-Mancera
Email: floresmancer@wisc.edu
Member of the Calibration Lab since 2021
Ultra-high dose rate (UHDR) beams are a promising technique for healthy tissue sparing. In comparison to conventional radiotherapy, UHDR beams could improve the clinical gain. Although the biological processes that explain this phenomenon are still unclear, it is certain that it is observed at high mean dose rates (≥ 40 Gy/s). However, the absolute dose determination is mostly constrained to the use of passive dosimeters such as alanine and radiochromic film, which have limited real-time dose monitoring. The response of charge-based detectors in the UHDR regime is compromised due to ion recombination, electrometer limitations, or both. The goal of my project is to develop a real-time dosimetry system for UHDR beams from pulse-by-pulse analysis using commercially available ion chambers. The pulse-by-pulse analysis will provide a real-time visualization of beam pulses, the discrimination of the induced current only due to electrons, and intra-pulse dose analysis.
Investigator: Sean Jollota
Email: jollota@wisc.edu
Member of UWMRRC since 2020
In the early 2000s, the use of alpha-emitting radionuclides for therapeutic applications experienced a resurgence due to advancements in Radiopharmaceutical Therapy (RPT). In RPT, solution-based radionuclides are injected into patients and guided to the treatment site via targeting molecules. The activity of these sources is measured prior to treatment, and computational methods are employed to convert this activity into absorbed dose following injection or insertion.
This conversion process is currently being verified by several National Metrology Institutes (NMIs), with a focus on validating nuclear decay and emission data. The accuracy of this conversion also depends on the radiation transport methods and parameters used in the computational models. Recently, there has been growing interest in the nuclear medicine community in establishing a traceability chain, similar to those used in external beam radiation therapy (EBRT). This traceability chain would include physical measurements of both the activity and the absorbed dose.
The goal of this project is to develop a standardization procedure that validates the dosimetry workflow of RPT through physical measurements of both activity and absorbed dose for a complex decay-chained alpha-emitting radionuclide, 225Ac.
(a)
(b)
Figure 1: (a) Extrapolation chamber designed and used for absorbed dose measurements of 225Ac and (b) printed circuit board collecting electrode that is used for parallelism alignment as well as measurement of ionization in air from the alpha and beta particles emitted from the 225Ac decay chain.
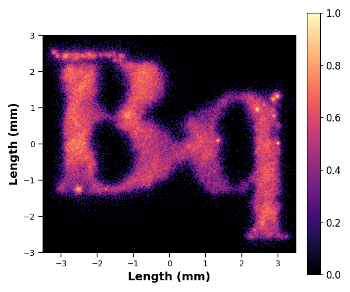
Investigator: Kelly McElvain
Email: kmcelvain@wisc.edu
Member of the Calibration Lab since 2023
Phantoms are indispensable tools in medical physics. They are generally used to simulate biological tissues or liquid water for applications such as dose verification, quality assurance (QA), and evaluation of medical imaging and therapy systems. Phantoms must accurately represent the physical and radiological properties of the material they are intended to simulate. Measurable characterization parameters include physical density, effective atomic number, electron density, and linear attenuation coefficients (LACs). These parameters help scientists appropriately select materials for their phantoms for their desired application. Well-established recommendations, such as The International Commission on Radiation Units and Measurements (ICRU) Report 44, facilitate this process. This report describes appropriate tissue substitutes for radiation dosimetry and measurements.
However, the extent to which a phantom will accurately represent a material is dependent on several factors including beam energy, geometry, and setup. For instance, a material that is water-equivalent at one energy may not be water-equivalent at another. This leads us to ask the following question: With so many commercially available materials for phantom production, how can we assist scientists in evaluating them to determine the most suitable one(s) for their experimental needs? This study aims to address that question for x-ray physics applications. X-ray attenuation properties of the several commercially available tissue- and water-mimicking solid phantoms were evaluated, relative to liquid water. The setup used for this this experiment is shown in Figure 1. Relative measurements were collected using an ion-chamber and electrometer pair. The water tank used for these experiments was designed by a former Cal Lab student, Michael Lawless, with the help of UWADCL staff. This setup was used for relative attenuation determinations across 50 to 250 kVp tube potentials. At higher energies, from 6 MV to 15 MV, percent depth dose curves (PDDs) will be used to more accurately characterize the relative attenuation of the solid phantom materials.

Investigator: Karen Rex Pius Vincent, M.S
Email: rexpiusvince@wisc.edu
Member of Calibration Lab since 2022
Primary standards for radiation measurement provide the highest accuracy, reduced measurement uncertainty, and traceability, ensuring consistency and reliability in medical applications such as brachytherapy. These standards are often established through absolute measurements, where quantities are determined without a calibration coefficient. Specifically, the air kerma rate can be measured absolutely with either a free-air chamber or a known-volume ionization chamber, with the latter used to measure higher energy photons (above 300 keV). However, in the U.S, only an interim primary standard currently exists for the air kerma strength (air kerma rate in vacuo) of HDR 192Ir brachytherapy seeds. This standard requires a calibration coefficient interpolated between two NIST-traceable beams (M250 and 137Cs) to match the effective energy of HDR 192Ir brachytherapy (397 keV). Therefore, this work aims to establish ionization chambers with precisely determined charge-collecting volumes for absolute measurements and potentially a new primary standard for HDR 192Ir air kerma strength.
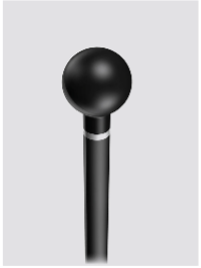
Investigator: Jacob Lambeck
Email: jlambeck@wisc.edu
Member of Calibration Lab since 2019
Absolute measurements of radiation dose have the potential to have lower uncertainties than more common relative measurement methods. However, due to difficulties in construction, their usage is generally limited to standards labs and thus their developments haven’t followed the advancements in clinical treatment methods. As treatment fields in radiation therapy have become more spatial heterogeneous, absolute dosimeters have generally remained only measuring at a single point, although attempts have been made at a line of measurements.
My research aims to develop and construct the first absolute dosimeter that can measure in multiple dimensions at once to give spatially dependent dose information. Primary concerns are the size of each calorimeter voxel, as smaller voxels allow for more fine spatial resolution, as well as keeping each voxel thermally independent so radiation dose deposited in one voxel does not affect the signal of neighboring voxels. The design of each voxel, as well as the array itself, is based off Monte Carlo and heat transfer simulations, machining limitations, material availability.
Investigator: Jordan Teague
Radioembolization therapy or selective internal radiation therapy (SIRT) is an advanced form of cancer treatment that combines radiation therapy and embolization to target liver tumors. This minimally invasive procedure involves injecting tiny radioactive beads, known as microspheres, directly into the arterial blood vessels feeding the tumor.
90Y microsphere treatment can be improved by reducing the impact of respiratory motion, dose calculation methods, and partition methods on dose estimate uncertainty. This project is a collaboration between the UW-MRRC and the Radiological Engineering and Design (RED) laboratory to develop methods for improving 3D personalized dosimetry for 90Y-microsphere liver treatment. The research approach consists of the development of a deformable phantom and reducing the impact of motion on 90Y-PET and SPECT.
Past Student Research Projects
aewalter2@wisc.edu
Member of the calibration lab since 2016
The Xoft Axxent® electronic brachytherapy source is a miniature, low energy and high dose rate x-ray tube that operates at tube potentials ranging from 40 to 50 kVp. Previous works have characterized the older, water cooled S7500 Axxent® sources, which were water cooled. A newer S7600 source has recently been introduced which used a Galden® coolant with a density of 1.72 g/cc. The introduction of this coolant can result in further filtration of the source spectrum and can influence the dose delivered from these sources compared to the S7500 sources.
The Axxent® sources can be used to treat a variety of cancers including breast cancer, skin cancer, and gynecological cancer. The treatment of gynecological cancers requires the use of a titanium cervical applicator. The use of this applicator causes further filtration of the source, resulting in a reduced dose rate of the source, a more monoenergetic spectrum, and a larger average energy. These modifications to the source output can influence the dose delivered from the source as well as influence the biological effects of the source. Thus, the purpose of this work is to dosimetrically and biologically characterize the newest Axxent® source model with and without the titanium cervical applicator present.
Measurements made with the Attix Free Air Chamber, TLD 100 microcubes, an A26 microionoization chamber, and EBT3 film were used to characterize these sources and benchmark Monte Carlo models in both TOPAS and EGSnrc for determining modified TG43 dosimetry parameters Additionally, clonogenic survival assays, comet assays, and chromosomal instability assays were performed using HeLa cells to determine the biological effects of these sources.
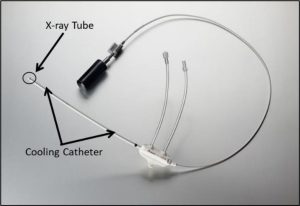
Investigator: Emily King, M.S.
eking24@wisc.edu
Member of the calibration lab since 2017
Energy dependent dosimeters used in clinics and research laboratories require calibration in a known radiation field of a closely matching energy to the radiation the dosimeters will be measuring. Primary standards labs, such as NIST in the United States, and secondary standards labs, such as the UW ADCL, have standard x-ray beams and radioactive sources like Cs-137 and Co-60 for the purpose of calibration. The standard x-ray beams established at NIST and matched at the UW ADCL have a maximum energy of 250 keV and the next energy step is Cs-137 which emits gamma rays with energy of 662 keV. There lies a gap in standard photon radiation between 250 keV and 662 keV which is an energy range that is increasingly becoming more commonly used. These applications in the higher energy range include radiobiology cabinet and conformal irradiators, Ir-192 brachytherapy sources, and the replacement of Cs-137 research irradiators with higher energy x-ray irradiators. The main goal of this work was to investigate the establishment of standard x-ray beams in this energy range and the development of the absolute dosimeters needed to establish them.
Outside of primary standards laboratories, a free-air chamber that could measure x-rays in the mediumenergy range did not exist prior to this work. The free-air chamber designed, built and benchmarked for this work can measure photons up to 320 keV. To measure higher energy x-rays, known-volume
ionization chambers were also established. These chambers typically require ultra-precise machining methods, but this work showed the possibility of establishing these chambers with micro-CT imaging and electric field modeling of commercial ionization chambers.
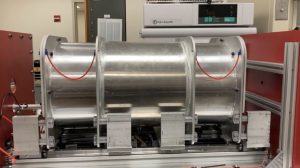
William Ferris, M.S.
wferris@wisc.edu
Member of the calibration lab since 2017
Intrafraction motion is motion of the patient that occurs during the course of a radiation therapy treatment delivery, such as breathing, bladder filling, or shifting on the treatment table. This motion decreases the quality of treatment by introducing uncertainties in the position of the target. The motion Synchrony system on the Radixact linear accelerator tracks and corrects for motion in real time during the treatment. Kilovoltage (kV) radiographs (2D) are acquired of the target location every 2- 10 s during treatment. In addition, the LEDs are placed on the patient’s chest, which are imaged with an optical camera mounted in the treatment room. The Synchrony system then builds a model to predict the target position using the information from the kV radiographs and the chest LEDs. This model is then used to steer the radiation to follow the respiratory motion of the tumor during treatment. This system became available for clinical use in 2019 and is growing internationally. The company who makes the Radixact, Accuray Inc., has a production facility in Madison and has an ongoing collaboration with UW-Madison. Our research is focused on quantifying the accuracy of the tracking and the dosimetric delivery using various devices.
Reed Kolany, M.S.
kolany@wisc.edu
Member of the calibration lab since 2018
Ultra-high dose-rate radiotherapy or FLASH therapy is a novel treatment technique which has gained traction in the field of medical physics and radiation therapy in recent years due to its capability to deliver treatments which result in similar local tumor control with increased normal tissue sparing at dose rates greater than 40 Gy/s. Since these normal tissue sparing effects are only seen using dose rates over 40 Gy/s, it is imperative to ensure the target areas are being irradiated at dose rates over this threshold. As a result, the goal of this project is to develop a dosimeter using ionization chamber and optical dosimetry methods that is capable of tracking the dose rate both spatially and over the duration of the treatment delivery for quality assurance purposes.
One of the novel approaches in this project is the use of light generated in plastic optical fibers alone to record the dose deposited at a temporal resolution high enough to capture individual beam pulses. As FLASH therapy treatments are delivered over a substantially lower time than conventional treatments, there are a fewer number of pulses delivered, and each pulse delivers considerably more dose, requiring the need for pulse-by-pulse monitoring of FLASH treatments, an approach not necessary in conventional radiotherapy.
The form this device will take will include two orthogonal arrays of optical fibers similar to the simple model below, and a small-gap ionization chamber for accurate and traceable dose measurements. Once completed, the device will be tested in both electron and proton beamlines and will output a 2D map of doses and dose rates indicating which areas received how much dose and how much of that dose was delivered over 40 Gy/s.
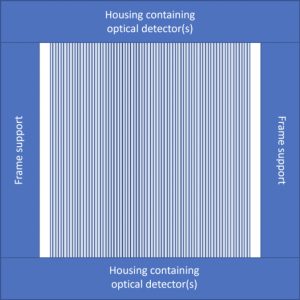
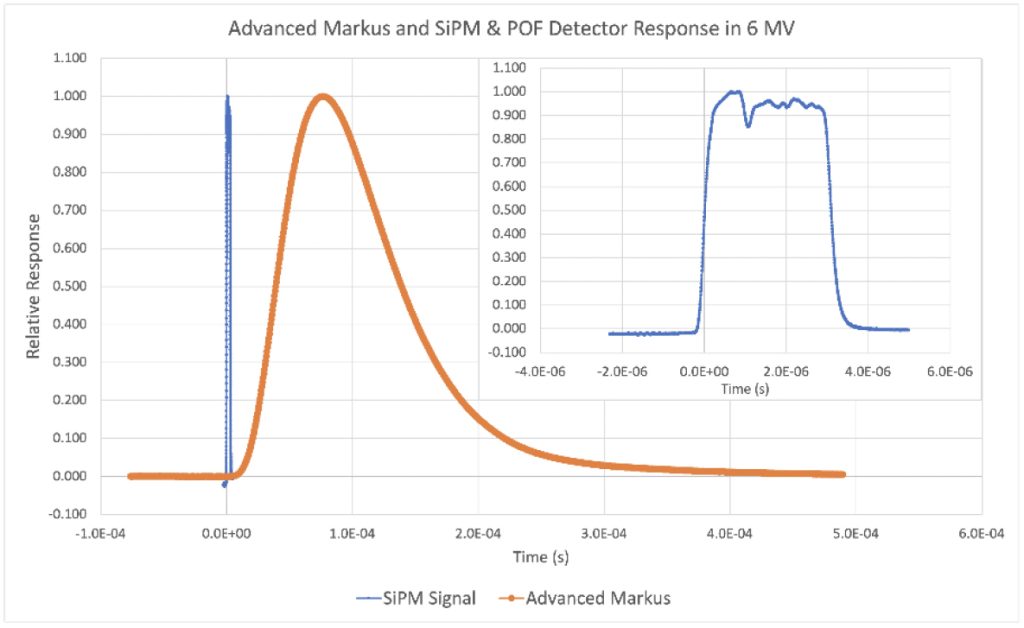
Andrew Bertinetti, M.S.
bertinetti@wisc.edu
Member of the calibration lab since 2018
Targeted radionuclide therapy (TRT) is a branch of radiation therapy which uses radioactive isotopes to deliver doses of radiation to targeted biological sites. Conformity is often achieved through the deposition of short-ranged charged particles, most often alpha and beta, in local tissue. Classically, complex biokinetic modeling of the patient, difficulties with accurately detecting short-range charged particles, and a lack of primary standards and adequate universal protocols for TRT dosimetry have led to large dosimetric uncertainties compared to other common forms of radiation therapy such as external beam radiation therapy (EBRT) and brachytherapy.
Recent advancements in medical imaging and computational efficiency have allowed Monte Carlo (MC) simulations to take on an integral role in personalized TRT dosimetry. MC codes such as EGSnrc, PENELOPE, and GEANT4 are already becoming increasingly popular for this purpose. However, the physics involved in the simulations rely on datasets compiled by NIST or other institutions. The validation of these codes in relation to absorbed dose to water (ADW) or tissue is essential for patient safety and clinical success in treatment. As such, it is necessary to establish easily adopted routines to benchmark these codes with physical dosimetry measurements.
Our research is focused on the dosimetry of beta-emitting TRT agents using common, readily available dosimeters, SPECT/CT imaging, and MC simulations. Phantom design and construction play integral roles for containment, dosimetry, and imaging purposes.
Ahtesham (Ash) Khan
akhan49@wisc.edu
Matriculated Fall 2018
Currently, there is a lack of traceability of absorbed dose to water in targeted alpha therapy (TAT). An absolute dosimeter is needed to directly measure absorbed dose from alpha-emitting radionuclides. Such a dosimeter can be used to determine a source-specific calibration coefficient (Gy/Bq) or can be used to validate commercial dose calculation softwares. Therefore, the goal of this work is to consider extrapolation chambers (EC) as primary standards for absorbed dose to water from alpha-emitting radionuclides. Absorbed dose to water will be measured using a planar (D400) and a segmented printed circuit board (PCB) extrapolation chamber. A pure alpha emitter such as 210Po will be initially used to evaluate the ECs before establishing traceability for clinically-relevant alpha sources such as 223Ra or 225Ac.
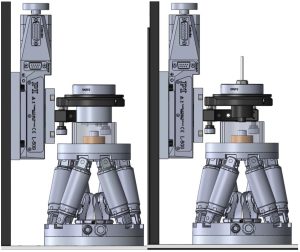
Jacob Lambeck,M.S.
jlambeck@wisc.edu
Member of the Calibration Lab since 2019
Following the guidelines of AAPM Task Group report 40, clinics must measure and verify the air-kerma strength of their brachytherapy sources prior to patient implantation. This procedure is usually done with an air-communication well-type ionization chamber and involves numerous correction factors. One such factor accounts for differences in the temperature and pressure conditions between when the well chamber was calibrated and when the brachytherapy source is being measured. For low-energy photon, low-dose rate sources, however, this correction is found to overcorrect at low ambient air pressures. To account for this, another altitude correction is applied, and the value of the correction is dependent on not only the ambient air pressure but is also source and chamber specific.
My work determined the corrections for two novel sources, the first source to use Cs-131 as its radioactive isotope, and a directional Pd-103 source. Through use of a diving bell-like pressure vessel, pressures at various altitudes were tested and the response of both sources in the two most commonly used well chambers were recorded. Fitting the chamber response versus pressure yields a pair of correction coefficients unique to that chamber model and the source that was tested, which can be used by clinicians when measuring their own sources to determine the altitude correction factor needed.
John Stasko, M.S.
jstasko@wisc.edu
Member of the calibration lab since 2019
Intensity-modulated radiation therapy (IMRT) is a common treatment technique that can produce superior dose distributions compared to conventional radiation therapy. The multi-leaf collimator (MLC), composed of many thin finger-like projections that can move into and out of the field, shapes the field into any desired configuration. The irregularly shaped fields and presence of the MLC leaves in IMRT add complexity to the dose calculation, so treatment planning systems make several concessions to avoid long computation times. These simplifications can lead to a difference between the dose measured during quality assurance and the calculated dose.
I have been working in collaboration with Dr. Sean Frigo from the Department of Human Oncology on designing a tool to analyze MLC position data from a DICOM RT plan. The program reads in the plan file and determines which points of the beam pass through which parts of the MLC leaves. Points are assigned to one of several transmission classifications based on this information and the assumptions of the treatment planning system. Using this data, we can compute several metrics and analyze their relationship with standard indicators of plan quality.

Karsten Wake, BS.
kkwake@wisc.edu
Member of the calibration lab since 2020
Gel dosimeters are made of a water equivalent material which undergoes chemical changes when exposed to ionizing radiation. The dose response, depending on the recipe of the gel, can include changes in opacity, density, and/or proton relaxation time, which is read out on optical computed tomography (OCT), x-ray CT, or magnetic resonance (MR), respectively. The dose response can be converted to dose, yielding a 3D dose distribution with very high spatial resolution.
3D dosimetry within an MRI-linac is uniquely challenging compared to conventional linacs due to the always-present magnetic field. Traditional dosimetry tools such as ion chamber and diode arrays can suffer from dose under- and over-response as well as orientation dependence. Gel dosimetry offers a useful alternative given the limitations of other 3D dosimetry methods and has already been shown to quantify effects between secondary electrons and magnetic fields, such as the electron return effect. This research seeks to further expand the applications of gel dosimetry with MR-linacs.
Nicholas (Nick) Nelson, M.S.
npnelson3@wisc.edu
Member of the calibration lab since 2018
The Dynamic Collimation System (DCS) is an energy layer-specific external collimation device designed to improve the lateral conformity in pencil beam scanning proton therapy (PBS-PT). The DCS consists of two pairs of nickel trimmers that rapidly and independently move and rotate to intercept scanning proton beams as they approach the target edge, that significantly reduces the adjacent normal tissue dose.
The DCS is a collaborative project between the University of Iowa, University of Wisconsin, and the Miami Cancer Institute’s proton therapy center, where the DCS is currently undergoing pre-clinical validation. As the DCS progresses towards clinical use, a significant amount of effort is being placed on experimentally validating the theorized dosimetric benefits of the DCS, mechanically integrating the DCS with the proton beamline control systems and commissioning a commercial treatment planning system to support DCS-based plan creation and optimization. My role on the project has been focused on developing and experimentally validating a Monte Carlo model of the DCS integrated with the proton beam at the Miami Cancer Center. This model has since served as the reference model to investigate mechanical and treatment planning-related aspects of the DCS implementation.
Riley C. Tegtmeier, M.S. candidate
rtegtmeier@wisc.edu
Member of the Calibration Lab since 2020
The focus of modern-day radiotherapy on highly specialized treatments to increase precision and visualization of target volumes has led to the development and improvement of image-guided radiotherapy (IGRT), in which patients are image before or during treatment delivery. These images are registered with reference images obtained during the treatment planning process to improve tumor localization and patient positioning to minimize the effects of intrafractional motion on geometric accuracy. However, in many instances, simply repositioning the patient is not sufficient to fully correct for certain anatomical changes over the course of treatment delivery, such as variations in the target volume and organs at risk (OAR) due to patient weight loss or tumor shrinkage. Adaptive radiotherapy (ART) refers to modifying the original treatment plan (refining contours, adapting dose prescription, etc.) during the course of therapy in response to these changes. For both IGRT and ART implementation to be effective, the performance of the imaging systems used to obtain the required volumetric information must be of sufficient quality to delineate anatomic structures as needed.
The Radixact helical tomotherapy treatment system consists of both an MVCT imaging system and a novel kVCT imaging system mounted orthogonal to the MV beam. Either imaging system can be utilized in IGRT and/or ART implementation. The purpose of this work is to characterize the image-quality performance of these systems and investigate how this performance may impact fidelity in the imageguided and adaptive processes depending on the modality used.